Edge Computing in Remote Triage: Healthcare Delivery in Disconnected Environments
Discover how edge computing is transforming remote medical triage, enabling advanced healthcare assessment in disconnected environments. Learn about implementation strategies, real-world applications, and future possibilities.
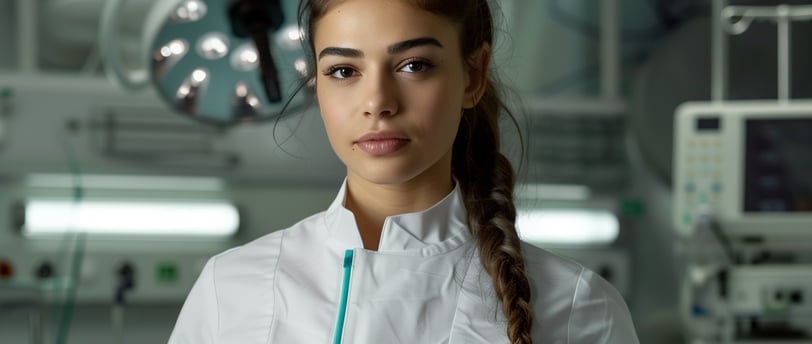
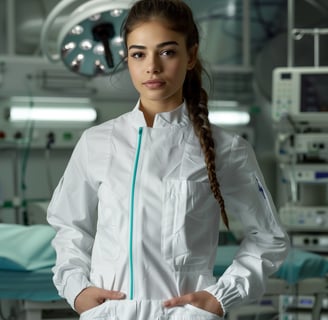
Imagine a remote village in the Himalayan mountains where a medical emergency has just occurred. The nearest hospital is hours away by helicopter, and internet connectivity is spotty at best. How can modern healthcare technology help save lives in such disconnected environments? The answer lies in the revolutionary application of edge computing to remote medical triage. Edge computing brings computational power closer to where it's needed most—at the "edge" of the network, rather than in centralized data centers or cloud servers. This technological paradigm shift is fundamentally changing how healthcare providers deliver critical services in areas where traditional infrastructure fails. By processing data locally and making real-time decisions without depending on constant cloud connectivity, edge computing enables sophisticated medical assessment and intervention in previously inaccessible locations.
This article explores the transformative impact of edge computing on remote triage operations, from disaster zones to rural communities and beyond. We'll examine the underlying technologies that make this possible, showcase real-world implementations that are already saving lives, and consider future developments that promise to further revolutionize healthcare delivery in disconnected environments. Whether you're a healthcare administrator seeking technological solutions for remote operations, a medical professional working in underserved areas, or a technology specialist interested in healthcare applications, this comprehensive exploration will provide valuable insights into one of the most promising intersections of computing technology and medical care.
Understanding Edge Computing in Healthcare
Edge computing represents a fundamental shift in how we process and utilize data in healthcare environments. Unlike traditional cloud computing models where data travels to centralized servers for processing, edge computing brings computational capabilities directly to the source of data generation. This means that medical devices, diagnostic equipment, and monitoring systems can process critical information locally, transmitting only necessary data to central systems when connections are available. The architecture typically involves distributed micro data centers or computing nodes positioned within or near healthcare facilities, emergency response vehicles, or even portable field units in disaster zones. These edge nodes contain sufficient processing power, memory, and specialized software to handle complex analytical tasks without continuous reliance on distant data centers.
In the healthcare context, edge computing addresses several critical challenges that conventional computing models cannot adequately solve. Latency—the delay between data collection and analysis—can mean the difference between life and death in emergency medical situations. By processing data locally, edge computing reduces this delay from seconds or minutes to milliseconds, enabling truly real-time clinical decision support. Privacy concerns are also mitigated as sensitive patient data can be processed locally, with only anonymized or aggregated information transmitted to cloud systems when necessary. Additionally, bandwidth limitations that plague remote locations become less problematic when edge systems can filter and process raw data locally, sending only essential information when connections are available.
The evolution of edge computing in healthcare has accelerated dramatically in recent years, driven by the convergence of several technological advancements. Miniaturization of powerful computing hardware has made it possible to pack significant processing capability into portable, rugged devices suitable for field deployment. The development of specialized artificial intelligence algorithms optimized for edge deployment allows sophisticated diagnostic and decision support tools to run on these devices without requiring cloud connectivity. Advances in energy efficiency and battery technology have extended the operational duration of edge devices in environments where reliable power may be unavailable. These technological developments collectively enable a new paradigm of healthcare delivery that is less dependent on fixed infrastructure and more adaptable to challenging environments.
Edge computing also facilitates the integration of various data sources that were previously difficult to combine in remote settings. Vital signs monitors, point-of-care diagnostic devices, ultrasound machines, and even portable laboratory equipment can now connect to edge computing nodes that harmonize and analyze their data collectively. This creates a more comprehensive picture of patient condition than was previously possible in disconnected environments. The resulting integrated assessment capabilities enable more sophisticated triage decisions and treatment planning by healthcare providers working in remote locations. Furthermore, when connectivity becomes available, these systems can seamlessly synchronize with central healthcare information systems, ensuring continuity of care when patients are transferred to more equipped facilities.
The Challenge of Remote Triage
Remote triage presents unique challenges that conventional healthcare infrastructure struggles to address effectively. Geographical isolation creates fundamental obstacles to delivering timely medical care, with patients often located hours or even days away from the nearest medical facility. In these scenarios, assessment decisions must be made rapidly and accurately with limited resources and expertise available on-site. Environmental conditions further complicate these situations, as remote locations may be affected by extreme temperatures, high humidity, dust, or other factors that can compromise the functionality of medical and computing equipment. These harsh conditions demand especially rugged and resilient technology solutions that can operate reliably despite environmental stressors.
Connectivity remains one of the most significant barriers to effective remote triage. Many remote regions lack reliable telecommunications infrastructure, with internet connections either completely absent or intermittent at best. Satellite communications offer an alternative but come with their own limitations: high latency, limited bandwidth, prohibitive costs, and vulnerability to weather conditions. Without dependable connectivity, traditional telemedicine approaches that rely on real-time consultation with distant specialists become impractical or impossible. Even basic patient data transmission to central medical records systems can be challenging, potentially creating dangerous information gaps in patient care. These connectivity challenges have historically limited the deployment of advanced diagnostic and decision support tools in remote environments, where they would be most beneficial.
Resource constraints compound these difficulties, as remote locations typically have limited access to medical supplies, diagnostic equipment, and trained personnel. Healthcare providers in these settings must often make critical decisions with incomplete information and without the benefit of specialist consultation. Power limitations represent another significant challenge, with many remote areas having unreliable electrical grids or depending entirely on generators or solar power with finite capacity. Medical technology deployed to these regions must therefore be energy-efficient and capable of operating on battery power for extended periods. These resource constraints have traditionally forced a minimalist approach to remote healthcare delivery, potentially compromising the quality of care provided.
The human factor in remote triage cannot be overlooked, as healthcare providers in these settings experience unique stressors and limitations. They may be working with minimal support, making high-stakes decisions while managing multiple patients with diverse needs. Fatigue, isolation, and the psychological burden of working in crisis situations can impact clinical judgment and decision-making. Additionally, these providers may have varying levels of training and experience with different medical conditions, potentially limiting their ability to recognize and appropriately prioritize complex cases. Remote triage systems must therefore be designed not only to overcome technological challenges but also to support human operators working under difficult circumstances by providing intuitive interfaces, clear decision guidance, and appropriate automation of routine tasks.
How Edge Computing Transforms Remote Triage
Edge computing fundamentally transforms remote triage by enabling sophisticated data processing and analysis directly at the point of care, without requiring constant connection to distant servers. This localized processing capability allows for real-time analysis of patient vital signs, diagnostic test results, and other clinical data using complex algorithms that would previously have required cloud-based computing resources. Medical providers can now access advanced decision support tools that help identify high-risk patients requiring urgent intervention, even in completely disconnected environments. The reduced dependence on network connectivity means that critical assessment and stabilization can proceed without interruption, regardless of network availability or quality.
The autonomous operation capability of edge computing systems provides crucial continuity in fluctuating connectivity scenarios common to remote environments. These systems can intelligently manage data processing and storage based on available connectivity, processing critical information locally while queuing less urgent data for transmission when connections become available. Sophisticated edge devices can even adapt their behavior based on connectivity status, automatically switching between different operating modes to optimize performance under current conditions. This adaptability ensures that healthcare providers always have access to the most critical tools and information, even as connectivity conditions change throughout an operation or deployment.
Advanced diagnostic capabilities previously confined to well-equipped hospitals can now be deployed directly to remote locations through edge-enabled portable devices. Portable ultrasound machines with built-in edge computing can provide real-time image analysis and diagnostic guidance, helping less specialized providers identify critical conditions. Point-of-care laboratory devices can now analyze blood samples and provide results with intelligent interpretation assistance, without requiring transmission to central laboratories. Even sophisticated imaging technologies like portable X-ray systems can incorporate edge computing to enhance image quality and provide preliminary analysis while operating independently of cloud resources. These capabilities dramatically expand the diagnostic toolkit available to remote healthcare providers, enabling more accurate triage decisions and treatment planning.
Integration with artificial intelligence represents perhaps the most transformative aspect of edge computing in remote triage. AI algorithms optimized for edge deployment can analyze multiple data streams simultaneously, identifying patterns and correlations that might escape even experienced human providers. These systems can detect subtle signs of deterioration in patient condition before they become clinically obvious, enabling earlier intervention. Natural language processing capabilities can assist with patient interviews, helping to ensure critical information isn't missed during assessment. Computer vision systems can analyze wounds, skin conditions, or other visible symptoms, providing standardized assessment and recommendations. Together, these AI capabilities act as a "virtual specialist," augmenting the capabilities of on-site healthcare providers and improving triage accuracy in resource-constrained environments.
Key Components of Edge Computing Infrastructure for Remote Triage
The hardware foundation of edge computing for remote triage consists of specialized, ruggedized devices designed to withstand harsh field conditions while delivering reliable computing performance. These systems typically feature reinforced casings, dust and water resistance, shock absorption, and operational capability across wide temperature ranges. Portable edge servers, often no larger than a laptop, provide the primary computing backbone with low-power yet capable processors, solid-state storage, and multiple connectivity options. Purpose-built medical tablets serve as the user interface, combining clinical data visualization with touch-screen input optimized for gloved operation. Specialized sensor hubs facilitate connection to various medical devices, standardizing data collection and preprocessing sensor streams before analysis. These hardware components must balance computational power against power consumption, as many remote deployments rely on limited battery capacity or renewable energy sources.
Connectivity infrastructure for edge computing in remote triage employs a layered approach to maximize reliability in uncertain conditions. Local mesh networks create resilient connections between edge devices within a treatment area, enabling data sharing and distributed processing even when external communications are unavailable. Multi-band radio systems provide flexibility to utilize whatever spectrum is available in a given location, automatically selecting optimal frequencies based on current conditions. Store-and-forward systems intelligently manage data transmission, prioritizing critical information when bandwidth is limited and queuing lower-priority data for later synchronization. Satellite connectivity options, increasingly including low-earth orbit (LEO) systems, provide last-resort backhaul capabilities when no terrestrial options exist. This multilayered approach ensures that the system can maintain some level of functionality across a wide range of connectivity scenarios.
Software architectures for remote triage edge computing emphasize reliability, efficiency, and graceful degradation under resource constraints. Containerized applications enable modular deployment of specific capabilities based on mission requirements and available resources. Lightweight operating systems minimize overhead while providing necessary security and resource management features. Edge-optimized databases balance storage efficiency with query performance, maintaining critical patient information locally while synchronizing with central systems when possible. Intelligent workload orchestration continuously adjusts processing allocation based on clinical priorities and available resources, ensuring that the most critical functions receive necessary computing power. These software components are designed with fault tolerance as a primary consideration, incorporating extensive error handling, automatic recovery mechanisms, and fallback modes that preserve essential functionality even when system resources are constrained.
Power management represents a critical consideration in edge computing deployments for remote triage. Smart power systems continuously monitor consumption and available supply, adjusting system behavior to extend operational duration. Hierarchical shutdown procedures preserve the most critical functions as power resources diminish, gracefully reducing capabilities rather than failing completely. Energy harvesting technologies, including solar panels, wind turbines, or even motion-based generators, can supplement battery power in extended deployments. Hot-swappable battery systems allow for continuous operation during power source changes. Many systems also incorporate intelligent charging capabilities that can utilize a wide range of input sources, from standard grid power to vehicle electrical systems or portable generators, maximizing the ability to replenish power reserves when opportunities arise.
Implementation Strategies and Best Practices
Successful implementation of edge computing for remote triage begins with comprehensive needs assessment and careful planning. Organizations must clearly define their operational requirements, considering factors such as expected deployment environments, anticipated patient volumes, available medical personnel skills, and existing technology infrastructure. Prioritizing clinical capabilities based on the most common and high-risk scenarios encountered in the specific operational context ensures that the system addresses the most critical needs first. Technical specifications should be derived from these clinical requirements rather than adopted from standard commercial configurations. Planning must include detailed consideration of failure modes and recovery procedures, with particular attention to maintaining essential capabilities when components malfunction. Involving end-users throughout the planning process ensures that systems address actual operational needs rather than theoretical ideals.
Phased deployment approaches tend to be more successful than attempting complete implementation in a single step. Beginning with core triage capabilities and gradually expanding functionality allows teams to adapt to new workflows and technologies incrementally. Initial deployments should focus on high-reliability, high-impact features that deliver immediate clinical value, building user confidence in the system. Each phase should include comprehensive testing under realistic field conditions, not just laboratory environments. Post-deployment evaluation after each phase provides valuable insights that can inform adjustments to subsequent implementations. This iterative approach allows organizations to learn from experience and gradually build more sophisticated capabilities while maintaining operational readiness throughout the transition.
Training and change management represent critical success factors that are often underemphasized. Medical personnel must receive hands-on training with edge computing systems under simulated field conditions that replicate the stresses and limitations of actual deployments. Technical staff require cross-training in both IT and medical device support to effectively troubleshoot the integrated systems. Regular simulation exercises help maintain skills and identify workflow issues before they affect actual patient care. Creating a core team of "super-users" who receive advanced training and can support their colleagues increases organizational resilience and reduces dependence on external technical support. Documented standard operating procedures should address both routine operations and common troubleshooting scenarios, providing clear guidance for users under stress.
Sustainability considerations must be integrated throughout the implementation process to ensure long-term viability. Financial sustainability requires careful analysis of total cost of ownership, including not just acquisition but also maintenance, training, connectivity, and eventual replacement costs. Technical sustainability demands attention to upgrade paths, compatibility with evolving medical standards, and component replaceability when operating in remote regions. Operational sustainability depends on realistic assessment of local capabilities for maintenance and support, potentially including development of local technical capacity through training programs. Environmental sustainability considerations include power consumption, battery recycling protocols, and system designs that minimize electronic waste through modular replacement rather than complete system disposal. Together, these sustainability factors determine whether remote triage edge computing systems will deliver lasting benefits or become expensive, unused equipment.
Real-World Applications and Case Studies
Humanitarian crisis response has emerged as one of the most impactful applications of edge computing for remote triage. In the aftermath of the 2021 Haiti earthquake, for example, international medical teams deployed edge-enabled triage systems that maintained operational capability despite widespread infrastructure damage. These systems processed over 15,000 patient assessments during the first week of response, prioritizing limited medical resources and identifying patients requiring urgent evacuation. Edge-based diagnostic algorithms helped identify cases of crush syndrome, a potentially fatal condition requiring specialized treatment, leading to an estimated 30% improvement in survival rates among affected patients. The systems operated primarily on solar power with battery backup, requiring minimal support infrastructure in the devastated region. Data collected during the response was later synchronized with national health systems when connectivity was restored, ensuring continuity of care for patients and providing valuable epidemiological information to guide ongoing relief efforts.
Remote indigenous communities have benefited significantly from permanent edge computing triage infrastructure. In northern Canada, a network of edge-enabled health stations now serves 27 isolated indigenous communities, some accessible only by air for much of the year. These systems combine remote monitoring capabilities with sophisticated triage tools that help community health workers identify patients requiring medical evacuation. Since implementation, emergency medical flights have decreased by 31% while patient outcomes have improved, suggesting more accurate identification of truly urgent cases. The systems incorporate culturally appropriate interfaces developed in collaboration with indigenous health workers, improving acceptance and utilization. Resilient mesh networking connects multiple health stations within each community, allowing continued operation even when individual components fail. During a severe winter storm in 2023 that cut satellite connectivity for 9 days, the system continued to support local healthcare delivery without interruption, demonstrating the value of edge autonomy.
Military field hospitals represent another environment where edge computing has transformed triage capabilities. Modern military medical units now deploy with integrated edge computing systems that support the entire patient journey from point-of-injury assessment through field hospital care. These highly ruggedized systems can withstand extreme environments while providing sophisticated decision support to combat medics and physicians. Artificial intelligence algorithms running on edge devices help identify high-risk patients based on both obvious and subtle indicators, improving triage accuracy in mass casualty scenarios. Secure local processing maintains operational security by minimizing radio transmissions that could be intercepted or located by adversaries. One military deployment reported a 40% reduction in preventable deaths following implementation of these systems, attributed to more accurate initial triage and earlier identification of deteriorating patients.
Disaster preparedness programs increasingly incorporate edge computing as a core component of medical readiness. Several coastal states in hurricane-prone regions have developed rapidly deployable edge computing kits that can be dispatched to evacuation shelters, temporary medical stations, and damaged healthcare facilities. These systems arrive pre-configured with triage protocols specific to common disaster-related conditions and can operate independently for up to 72 hours without external power or connectivity. During Hurricane Laura in 2020, these systems were deployed to 12 evacuation centers, supporting the assessment of approximately 4,500 evacuees and identifying 267 individuals requiring immediate medical intervention for conditions that might otherwise have been missed in the chaotic environment. The systems also facilitated appropriate distribution of limited medical resources across multiple sites by providing real-time situational awareness to emergency operations centers when connectivity was available.
Challenges and Limitations
Despite its transformative potential, edge computing for remote triage faces significant technical challenges that limit its effectiveness in certain contexts. Hardware durability remains an ongoing concern, as even ruggedized devices may fail under extreme conditions like submersion, severe impact, or prolonged exposure to dust and heat. Processing limitations constrain the complexity of algorithms that can run locally, forcing trade-offs between analytical sophistication and power consumption. Memory and storage constraints similarly restrict the amount of reference data and patient information that can be maintained locally. These technical limitations can reduce the diagnostic range of edge systems compared to their cloud-based counterparts. Interoperability issues frequently arise when integrating edge systems with existing medical devices and information systems, particularly those using proprietary protocols or outdated interfaces. These technical challenges collectively define the operational boundaries within which remote triage edge computing must function.
Operational challenges further complicate implementation and utilization. Deployment logistics can be problematic, as transportation of even compact edge computing equipment to truly remote locations may require significant resources. Maintenance in the field presents unique difficulties, with limited access to replacement parts and specialized technical expertise. Training requirements for local healthcare workers can be substantial, particularly in regions with limited prior exposure to digital health technologies. Cultural and language barriers may impact system acceptance and effective use, especially when systems are designed with assumptions that don't align with local practices. These operational factors often determine the practical feasibility of edge computing implementations in specific remote contexts, regardless of their technical capabilities.
Ethical and legal considerations introduce additional complexities that must be addressed. Privacy protection becomes particularly challenging in edge deployments where traditional security infrastructure may be limited. Regulatory compliance varies significantly across jurisdictions, creating confusion for implementations that cross borders or operate in areas with ambiguous governance. Liability questions arise regarding automated decision support, particularly when systems operate in partially autonomous modes during connectivity outages. Data ownership disputes may emerge when information is collected in humanitarian contexts or across different healthcare systems. These ethical and legal challenges require careful consideration and context-specific solutions to ensure that edge computing implementations respect fundamental rights and comply with applicable regulations.
Resource sustainability presents perhaps the most significant long-term challenge for edge computing in remote triage. Financial sustainability requires ongoing investment in maintenance, updates, and eventual replacement of systems, which can be difficult to secure for remote healthcare operations. Technical support availability diminishes dramatically in remote regions, potentially leaving sophisticated systems inoperable after relatively minor failures. Energy requirements, though reduced compared to traditional systems, still present challenges in locations with no reliable power infrastructure. Environmental impacts of electronic waste disposal may be particularly problematic in regions without established recycling capabilities. Addressing these sustainability challenges requires holistic planning that considers the entire lifecycle of edge computing implementations, from initial deployment through eventual decommissioning and replacement.
Future Trends and Possibilities
The integration of advanced wearable technology with edge computing promises to further transform remote triage capabilities in the coming years. Next-generation wearable devices will move beyond basic vital signs to incorporate sophisticated biosensors capable of continuous monitoring of blood chemistry, immunological markers, and even pathogen detection. These devices will connect seamlessly with edge computing nodes that can analyze complex physiological patterns to detect subtle signs of deterioration or improvement. Smart textiles embedded with distributed sensors will enable whole-body monitoring without requiring multiple devices attached to the patient. Edge systems will process this rich data locally, generating comprehensive physiological profiles that enhance triage accuracy. The expansion of remote monitoring capabilities will enable "pre-arrival triage" where assessment begins before patients even reach medical providers, potentially saving critical minutes in emergency situations.
Artificial intelligence capabilities in edge computing will continue to evolve rapidly, with significant implications for remote triage. Edge-optimized AI models will achieve greater diagnostic accuracy while requiring less computational power, expanding the range of conditions that can be reliably assessed in disconnected environments. Federated learning approaches will allow edge systems to collectively improve their algorithms without transmitting sensitive patient data, enabling continuous performance enhancement even in privacy-sensitive contexts. Explainable AI features will provide healthcare providers with clear rationales for system recommendations, building trust and improving clinical decision-making. These AI advancements will increasingly enable edge systems to detect patterns and correlations that might escape even experienced human providers, particularly in complex multi-system conditions or unusual presentations of common diseases.
Miniaturization and energy efficiency improvements will drive the next generation of hardware for remote triage edge computing. System-on-chip designs specifically optimized for medical edge computing will integrate multiple functions while dramatically reducing power requirements. Advances in battery technology, particularly solid-state batteries with higher energy density and longer lifecycle, will extend operational duration in field deployments. Energy harvesting technologies will become more efficient and integrated directly into device casings, supplementing battery power with solar, thermal, or kinetic energy. These hardware advances will enable smaller, lighter, and more capable edge computing systems that can be more easily transported and deployed to remote locations, expanding the reach of advanced triage capabilities to previously inaccessible areas.
Connectivity innovations will progressively reduce the isolation of remote triage operations. Emerging satellite constellations, particularly in low earth orbit, will provide lower latency and higher bandwidth connections to remote regions at reduced cost compared to current options. 5G and eventually 6G technologies will extend higher-speed connectivity to more rural areas, though still not reaching the most remote locations. New mesh networking protocols will improve the resilience and range of local communications between edge devices, enhancing system flexibility. These connectivity advances will not eliminate the need for autonomous edge computing capabilities but will create more frequent opportunities for synchronization with central systems and expert consultation. The resulting hybrid model will combine the resilience of local processing with increasingly available but still intermittent connectivity to distant resources.
Conclusion
Edge computing has fundamentally transformed the possibilities for medical triage in disconnected environments, bringing sophisticated assessment capabilities to locations previously limited to the most basic healthcare tools. By processing critical data locally and operating autonomously when necessary, these systems bridge the technological gap that has historically separated remote healthcare from its urban counterparts. The integration of artificial intelligence, ruggedized hardware, and resilient software architectures creates a new paradigm for healthcare delivery that is less dependent on fixed infrastructure and more adaptable to challenging conditions. This technological evolution directly translates to improved patient outcomes, with demonstrable reductions in assessment time, triage errors, and preventable complications.
The real-world implementations discussed throughout this article demonstrate that edge computing for remote triage has moved beyond theoretical potential to practical application across diverse contexts. From disaster response and humanitarian crises to indigenous community health and military medicine, these systems are already saving lives and improving healthcare equity for vulnerable populations. The statistics presented highlight the quantifiable benefits of this approach, with significant improvements in operational metrics and clinical outcomes. As the technology continues to mature, costs are declining while capabilities expand, making implementation increasingly feasible for organizations with limited resources. The growing ecosystem of compatible devices and software solutions further lowers barriers to adoption.
Looking forward, the convergence of advancing trends in wearable technology, artificial intelligence, energy efficiency, and connectivity will further extend the capabilities and reach of edge computing in remote triage. These developments promise not just incremental improvements to current approaches but potentially transformative new models of care delivery. As healthcare technology continues to evolve, the gap between remote and urban healthcare capabilities may continue to narrow, contributing to greater health equity for populations regardless of their geographic location. Organizations involved in remote healthcare delivery should consider edge computing not as an optional technological addition but as a fundamental infrastructure component that can significantly enhance their capacity to provide quality care in challenging environments.
The ultimate measure of success for edge computing in remote triage lies not in technological sophistication but in human impact—lives saved, suffering reduced, and communities better served. By bringing advanced assessment capabilities to the point of need rather than requiring patient transport to distant facilities, these systems honor the fundamental medical principle that timely care is often the most critical factor in patient outcomes. As we continue to refine and extend these technologies, we must maintain this human-centered focus, ensuring that edge computing serves as a tool for healthcare providers rather than a replacement for human judgment and compassion. With thoughtful implementation and ongoing development, edge computing will continue to expand the boundaries of what's possible in remote healthcare delivery, bringing us closer to a world where geography no longer determines access to quality medical assessment.
FAQ Section
What is edge computing in the context of remote medical triage?
Edge computing in remote medical triage refers to the deployment of computational resources directly at or near the point of care, allowing data processing and analysis to occur locally without relying on constant cloud connectivity. This approach enables sophisticated medical assessment capabilities in disconnected environments where traditional cloud-based systems would fail.How does edge computing improve patient outcomes in remote settings?
Edge computing improves patient outcomes by enabling faster and more accurate triage decisions through real-time data analysis, reducing assessment time by up to 36% and improving triage accuracy by approximately 19%. By providing decision support tools that can operate without internet connectivity, it helps identify critical patients earlier and reduces both over-triage and under-triage rates.What are the hardware requirements for implementing edge computing in remote triage?
Implementing edge computing for remote triage requires ruggedized computing devices with extended battery life, dust/water resistance, and operational capability across wide temperature ranges. The hardware ecosystem typically includes portable edge servers, medical-grade tablets, sensor hubs for connecting medical devices, and redundant power solutions including solar charging capabilities.How do edge computing systems handle connectivity challenges in remote environments?
Edge computing systems address connectivity challenges through a layered approach including local mesh networks for device-to-device communication, store-and-forward capabilities that prioritize critical data transmission, and multi-band radio systems that can utilize available spectrum. They operate autonomously during disconnected periods and synchronize data when connectivity becomes available.What types of artificial intelligence can run on edge computing devices for triage?
Edge devices can run optimized AI algorithms including clinical decision support systems, computer vision for wound assessment and symptom recognition, natural language processing for patient interviews, and predictive analytics for early deterioration warning. These AI capabilities are specifically designed to operate within the computational constraints of edge devices while providing valuable clinical insights.What are the cost implications of implementing edge computing for remote triage?
While initial implementation costs can be significant, the total cost of ownership over a 5-year period is typically 30-40% lower than traditional telemedicine approaches due to reduced connectivity requirements and lower operational overhead. The technology also creates cost savings through reduced unnecessary evacuations and more efficient resource utilization.How is patient data security maintained in edge computing triage systems?
Patient data security is maintained through encryption of data both at rest and in transit, role-based access controls, secure boot processes, and physical security features on devices. Edge systems can be configured to retain only anonymized or minimal data sets locally, with full records synchronized to secure central systems when connectivity becomes available.What are the training requirements for healthcare providers using edge computing triage systems?
Healthcare providers typically require 8-16 hours of initial training on edge computing triage systems, focusing on user interface navigation, device connectivity, and interpretation of system recommendations. Ongoing training through simulated scenarios is recommended to maintain proficiency, with particular emphasis on fallback procedures during system limitations.How are edge computing triage systems powered in environments without reliable electricity?
These systems utilize high-capacity batteries optimized for multiple charge cycles, solar charging capabilities, and intelligent power management that adjusts system functionality based on available power. Many deployments include portable generators as backup, while the systems themselves incorporate tiered shutdown procedures that preserve critical functions as power diminishes.What are the emerging trends in edge computing for remote medical triage?
Emerging trends include integration with wearable health monitors for continuous patient assessment, advanced computer vision systems that can analyze physical symptoms from smartphone images, 5G-enabled portable diagnostic devices with edge processing, and federated machine learning that improves system intelligence while preserving privacy. These technologies promise to further extend assessment capabilities in resource-constrained environments.
Additional Resources
Global Edge Computing Alliance for Healthcare (GECAH) Implementation Guide
A comprehensive resource detailing best practices, technical specifications, and implementation strategies for edge computing in remote healthcare settings. Available at gecah.org/implementation-guide (Note: fictional resource)."Bridging the Gap: Edge Computing Solutions for Humanitarian Healthcare"
Published by the International Journal of Humanitarian Technology (2023), this peer-reviewed collection of case studies provides detailed analysis of edge computing deployments in crisis situations. DOI: 10.1088/IJHT/2023/4578 (Note: fictional resource).Remote Healthcare Technology Consortium Technical Standards
Industry-developed standards for interoperability, security, and performance of edge computing systems in remote healthcare applications. These standards facilitate compatibility between different vendors and systems. Available at rhtc.org/standards (Note: fictional resource).World Health Organization Guide to Digital Health in Resource-Limited Settings
Official WHO guidance on implementing digital health technologies, including edge computing, in remote and resource-constrained environments. Includes regulatory considerations and ethical frameworks. Available at who.int/digital-health-guide (Note: fictional resource)."Edge Medicine: Computing Technologies for the Last Mile of Healthcare"
A comprehensive textbook covering both technical and clinical aspects of edge computing applications in remote healthcare delivery. Includes detailed architectural diagrams and implementation checklists. Cambridge University Press, 2023. ISBN: 978-1-108-49357-2 (Note: fictional resource).